
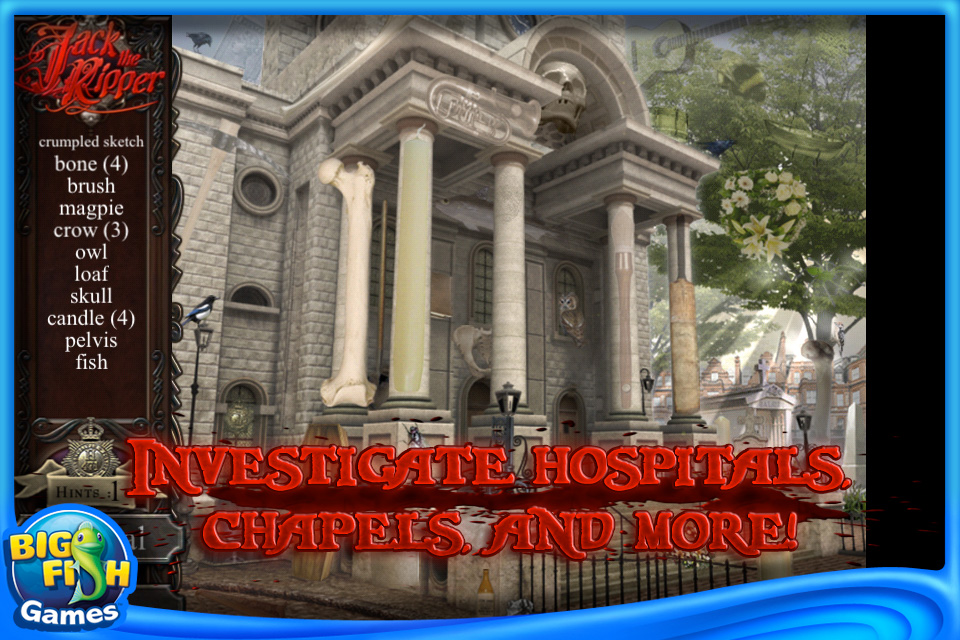
A series Anderson's VCUUV 0.61 _ The flapping frequency is below 1 Hz Yu's carangiform swimmer 0.8 _ Use three motors and the flapping frequency is 2 Hz Wen's carangiform swimmer 0.98 0.41 The flapping frequency is 1.8 Hz Hu's Essex G9 1.02 0.5 The flapping frequency is 1.3 Hz MIT compliant robot fish 1.1 0.7 The flapping frequency is 3 Hz Hu's Essex iSplash 11.6 0. 2) Turning Test: The second set of experiments was performed to test the turning performance of the robot fish. This research provides a guidance on the kinematic optimization and motor control of the undulatory robot fish. Moreover, the kinematics study has shown that the body curves of the robot fish were similar to the referenced body curves presented by the formula when using the sinusoidal pattern, especially the major thrust generation area. The over-cambered sinusoidal pattern was compromised in both metrics. Based on the experiment results, it is found that the sinusoidal pattern generated the largest average static thrust and steady cruising speed, while the triangular pattern achieved the best yaw stability. Three driving patterns of the motor are experimented to achieve three kinematic patterns of the robot fish, e.g., triangular pattern, sinusoidal pattern, and an over-cambered sinusoidal pattern. A dynamic model integrated with surrounding fluid is developed to predict the cruising speed, static thrust, dynamic thrust, and yaw stability of the robot fish. The robot fish consists of a wire-driven body and a soft compliant tail, which can perform undulatory motion using one motor. In this paper, we adopt a biomimetic untethered robot fish to study the kinematics of fish flapping. However, the reason why robot fish must follow this formula has not been fully studied. Recent state-of-art researches on robot fish focus on revealing different swimming mechanisms and developing control methods to imitate the kinematics of the real fish formulated by the so-called Lighthill's theory. Individually, miniature and low-cost underwater robots can also provide a platform for the study of 3D autonomy and marine vehicle dynamics in educational and resource-constrained settings. The robot is designed to form the basis for underwater swarm robotics testbeds, where low cost and ease of manufacture are critical, and 3D maneuverability allows testing complex coordination inspired by natural fish schools. We present several results on the robot, including: (i) quantified open-loop swimming characteristics (ii) autonomous behaviors using a pressure sensor and an IMU to achieve controlled swimming of prescribed trajectories (iii) feedback from an optic sensor to enable homing to a light source. This allows us to create a robot with multiple flapping fin propulsors that independently control robot motions in surge, heave, and yaw. A key aspect of the robot design that makes this possible is the use of low-cost magnet-in-coil actuators, which have a small profile and minimal sealing requirements.
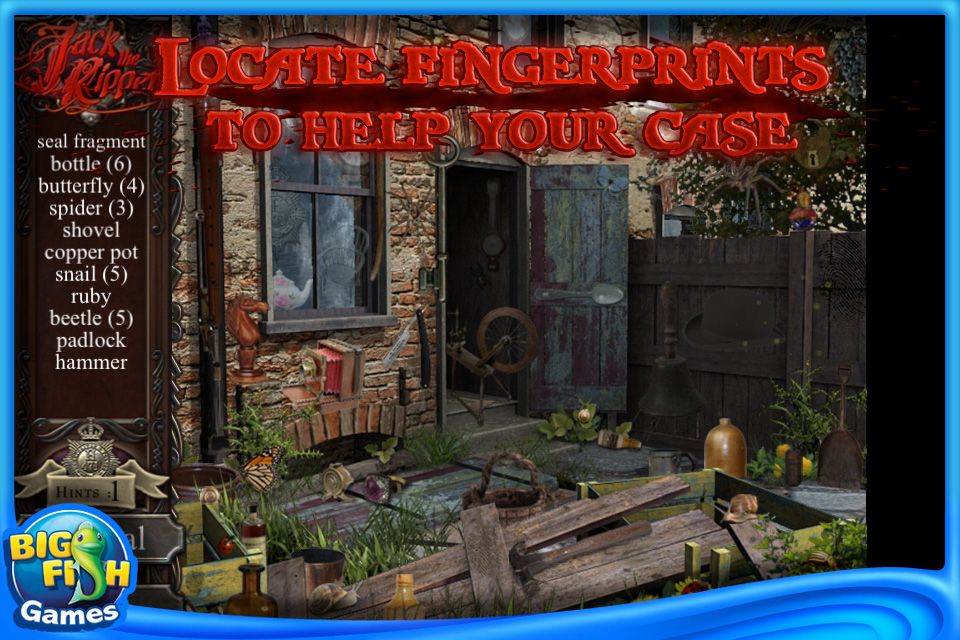
In this paper we present the design of a miniature (100 mm) autonomous underwater robot that is low-cost ($100), easy to manufacture, and highly maneuverable. Nanyang Arowana-like fish (NAF) consisted of a master controller which controlled different local controller which was used for controlling the actuators.
#BIG FISH ISPLASH SERIAL#
Joint level control is implemented via various means like layered control architecture consisting of three layers, namely, (1) cognitive layer, (2) behavior layer and (3) swim pattern layer, remote control, CPG control, serial communication, H ∞ control method, Computed Torque Method (CTM), radio-control, speed controller, fuzzy logic control method, Bio-inspired distributed control, sinusoidal motion control, pitch control mechanism, PID based controller to control the robot speed by varying the frequency of link oscillation, simplified wave control method, hierarchical control algorithm, approximate control algorithm and open loop control of links.
